
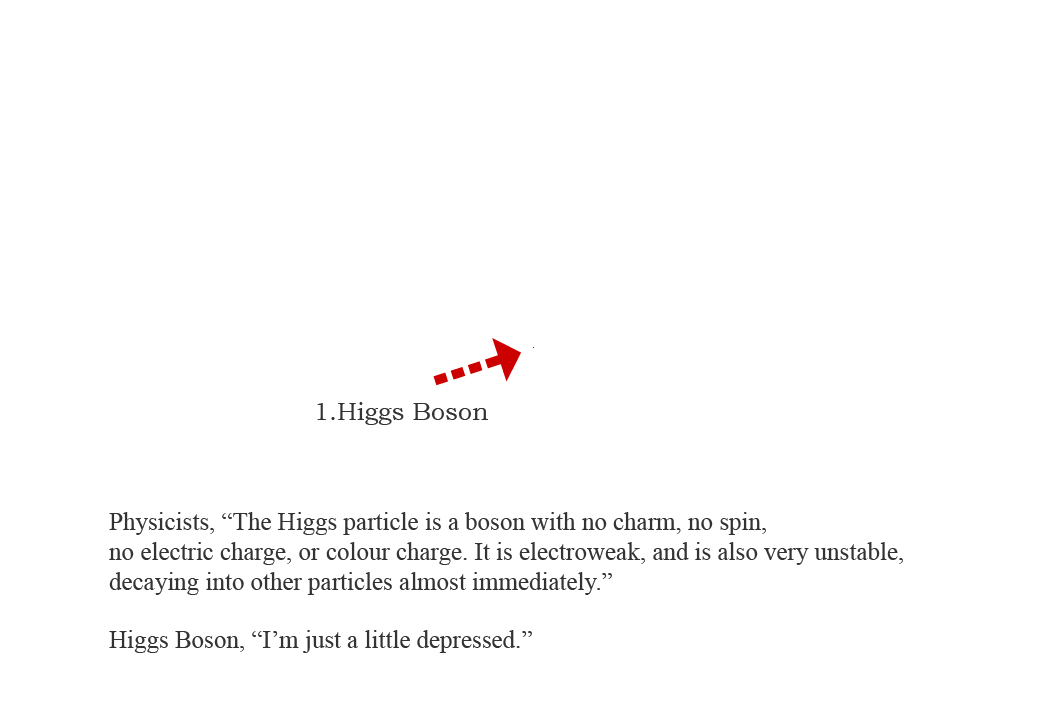
Scientists thus need some way of determining when a pair of photons (or four muons or a different final state that the Higgs decays into) is coming from a Higgs boson decay and when it’s not. Especially since the Higgs boson is only produced about once in a billion of these collisions. Simply seeing a pair of photons (one of the final states from the Higgs boson decay) is hardly any indication that the Higgs boson exists and is being produced in the experiment. The problem is that the particles that the Higgs decays into are the same kinds of particles that are copiously produced in particle collisions. Once the decay products have been detected, the next step is to determine whether we can say that the Higgs boson was produced. These traces have to be detected and precisely measured by particle detectors. The particles from the boson’s decay are the only traces that it leaves behind. So it is not possible to observe it directly. Given its lifetime, the Higgs boson almost immediately decays – or transforms – into other particles. Producing the new particle is only the first step, however. The first particle collider in history capable of producing Higgs bosons in significant numbers is the Large Hadron Collider (LHC), which started its high-energy collision programme in 2010. This large mass, combined with an extremely short lifetime (10 -22 seconds) means that the particle cannot be found in Nature – its existence can only be verified by producing it in the lab. With a mass of more than 120 times that of the proton, the Higgs boson is the second-heaviest particle known today. The Higgs boson was discovered, almost 50 years after first being proposed, by the ATLAS and CMS collaborations at CERN in 2012. The lower event in the ATLAS experiment shows a decay into four muons (red tracks) (Image: CMS/ATLAS/CERN) The top event in the CMS experiment shows a decay into two photons (dashed yellow lines and green towers). Candidate Higgs boson events from collisions between protons in the LHC.
